GREEN CONCRETE AND SUSTAINABILITY OF ENVIRONMENTAL SYSTEM
1.0 What is GREEN concrete?
Engineers and architects can compare materials and choose one that is more sustainable or specify a construction material in such a way as to minimize environmental impact? Recent focus on climate change and the impact of greenhouse gas emissions on our environment has caused many to focus on CO2 emissions as the most critical environmental impact indicator. Life Cycle Assessment (LCA) is the parameter; the construction industry should look into. LCA considers materials over the course of their entire life cycle including material extraction, manufacturing, construction, operations, and finally reuse/recycling.
2.0 Features of Green Concrete:
Where does the Carbon Dioxide come from in concrete?
There have been a number of efforts about reducing the CO2 emissions from concrete primarily through the use of lower amounts of cement and higher amounts of supplementary cementitious material (SCM) such as fly ash, blast furnace slag etc. CO2 emissions from 1 ton of concrete produced vary between 0.05 to 0.13 tons. 95% of all CO2 emissions from a cubic meter of concrete is from cement manufacturing. It is important to reduce CO2 emissions through the greater use of SCM.
i) Cement:
CaCO3 = CaO + CO2
ii) Aggregate:
iii) Resources:
Green Concrete:
Obtaining the most suitable mix based on the specification or suggesting improvements in the mix is to assist with the most suitable concrete for the project. The concrete which can fall in the category of green must have the following characteristics.
- Optimizes use of available materials
- Better Performance
- Enhanced cohesion workability / consistency
- Reduced shrinkage / creep.
- Durability – Better service life of concrete
- Reduced carbon footprint
- No increase in cost
- LEED India Certification
Green concrete mix is designed with the principle of “Particle-Packing Optimization” to meet requirements of plastic and hardened properties.
3.0 Materials for Green Concrete:
Green construction materials are composed of renewable, rather than non-renewable resources. Green materials are environmentally responsible because impacts are considered over the life of the product. Depending upon project-specific goals, green materials may involve an evaluation of one or more of the following criteria.
- Locally available: Construction materials, components, and systems found locally or regionally, saving energy and resources in transportation to the project site.
- Salvaged, re-furnished, or re-manufactured: Includes saving a material from disposal and renovating, repairing, restoring, or generally improving the appearance, performance, quality, functionality, or value of a product.
- Reusable or recyclable: Select materials that can be easily dismantled and reused or recycled at the end of their useful life.
Recycled materials that the Industry has found to perform favorably as substitutes for conventional materials include: fly ash, granulated blast furnace slag, recycled concrete, demolition waste, microsilica, etc. Generation and use of recycled materials varies from place to place and from time to time depending on the location and construction activity as well as type of construction projects at a given site. Following materials can be considered in this category and are discussed here.
a. Recycled Demolition Waste Aggregate
b. Recycled Concrete Aggregate
c. Blast furnace Slag
d. Manufactured Sand
e. Glass Aggregate
f. Fly ash
They are divided in cement, cementitious material, coarse and fine aggregate. Their definitions are as usual.
4.0 Coarse Aggregate:
Aggregate contents have direct and far-reaching effect on both the quality and cost of concrete. Unlike water and cement, which do not alter in any particular characteristic except in the quantity in which they are used, the aggregate component is infinitely variable in terms of shape, size and grading etc. With coarse aggregates graded infractions between 5mm and 40mm, differences in particle shape and surface texture affect the bulk void content and frictional properties of concrete. Generally the requirement of course aggregate in concrete is more than 50% as shown in figure 1. Similarly sand required is about 30%. They contribute in large quantity so its availability and effect on environment must be carefully examined. Following source of coarse aggregate are discussed: Figure 1 – Distribution of Ingredients in a typical Concrete Mix
- Fresh Local Aggregate
- Recycled Demolition Waste Aggregate
- Recycled Concrete Material (RCM)
- Blast Furnace Slag (BFS)
A) Fresh Local Aggregate:

B) Recycled Demolition Waste Aggregate:
Figure 3 – A typical Waste Dump on the Road sides
Therefore, for sustainability of resources, it is necessary that all waste must be scientifically managed. Waste Management is Collection, Transport, Processing, Recycling or disposal of waste materials. When analyzed, a typical waste product distribution in any solid waste dump is shown in figure 4. This waste distribution shows that there is about 50% demolition waste in the dump. In order to have sustainability of resources this demolition waste must be recycled and used.
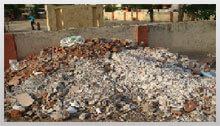
Figure 3 – A typical Waste Dump on the Road sides
Therefore, for sustainability of resources, it is necessary that all waste must be scientifically managed. Waste Management is Collection, Transport, Processing, Recycling or disposal of waste materials. When analyzed, a typical waste product distribution in any solid waste dump is shown in figure 4. This waste distribution shows that there is about 50% demolition waste in the dump. In order to have sustainability of resources this demolition waste must be recycled and used.

Figure 4- Typical Distribution of material in waste Dump lying on road side
Recycled Aggregate is produced from Broken Building Part called MALWA. Such demolition waste – MALWA, can be converted to Course aggregate. The Demolition waste can be broken into the pieces of approximately 20 & 10 mm size with the help of light crusher. Typical shape & color of recycled aggregate is shown in figure 5. This type of processing the waste will make the system Sustainable.
The physical and chemical properties of such recycled aggregate must be determined before use as given in chapter III. The properties of recycled aggregates will vary from place to place and from time to time. For example, the specific gravity of recycled aggregate may be less than fresh conventional aggregate because it may have mixture of materials.
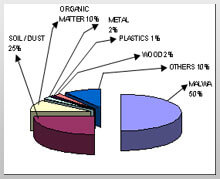
Use In General Purpose Concrete:
If properties of sample recycled aggregate are suitable then such aggregate can used in concrete mix. The concrete mix design can be done as of general method described earlier. The shape of slump cone of concrete with recycled aggregate is generally similar to conventional aggregate. The failure pattern of cubes / fracture mechanism is also similar to conventional cubes as shown in figure 6.
If properties of sample recycled aggregate are suitable then such aggregate can used in concrete mix. The concrete mix design can be done as of general method described earlier. The shape of slump cone of concrete with recycled aggregate is generally similar to conventional aggregate. The failure pattern of cubes / fracture mechanism is also similar to conventional cubes as shown in figure 6.
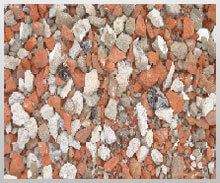
C) Recycled Concrete Material (RCM)

Crushed concrete’s physical characteristics make it a viable substitute for coarse aggregate however, its physical and chemical properties must be determined before use as given in chapter III. The properties of recycled aggregates will vary from place to place and from time to time. Such aggregate can be used in concrete mix or in Highways concrete construction similar to demolition waste aggregate concrete.
Figure 6 – Failure Pattern of Crushed Bricks made with Recycled Aggregate
D) Blast Furnace Slag (BFS):
Crushed Air-Cooled Blast Furnace Slag may be broken down as typical aggregate with the help of processing equipment to meet gradation specifications. Thus, blast furnace slag can be available as an aggregate as construction materials and acceptable as coarse or fine aggregate for use in green Concrete. A typical shape of ACBFS coarse aggregate is shown in figure 7.
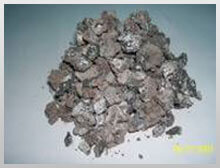
5.0 Fine Aggregate (Sand):
Following source of Fine aggregate are normally used. Some are discussed here:
- Fresh River Sand
- Manufactured Sand
- Recycled Glass aggregate.
- Blast Furnace Slag (BFS)
A) Manufactured Sand For Concrete:
Optimum Shape:
Figure 8 – Shape of Manufactured Sand (Enlarged view through Microscope)

Natural Sand Vs Manufactured Sand:
It has been proven that about 20kg of cement can be saved for every cubic meter of concrete that is made by replacing a poorly shaped aggregate with a cubical aggregate. In addition, both compressive strength and flexural strength are improved by using cubical aggregates, which also increases workability and reduces bleeding and shrinkage. The impact of the physical characteristics of the sand used in the concrete mix is even greater than that of the coarse aggregate fractions, both in the concrete’s plastic and hardened states
Recycled Glass Aggregate:
When glass is properly crushed, this material exhibits fineness modulus & coefficient of permeability similar to sand. It has very low water absorption. High angularity of this material, compared to rounded sand, enhances the stability of concrete mixes. Such material can be easily used in concrete construction as fine aggregate and give a better cohesive mix which will save on the consumption of cement.
Blast Furnace Slag (BFS):
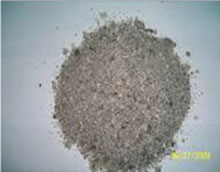
6.0 Cementitious materials – Fly Ash:
Fly ash particles are very smooth and quite spherical in shape. These particles range from 1 to 150 m in diameter. A typical shape of fly ash particles is seen in figure 10. Based on its composition, fly ash is classified into two groups: ASTM Class C or high calcium fly ash and ASTM Class F or low calcium fly ash are the two categories of fly ash.

Use of Fly ash & Economic Impact:
Advantages Of Using Fly Ash in Concrete:
- Utilization of fly ash as a part replacement of cement or as a mineral admixture in concrete saves on cement and hence the emission of CO2.
- Use of good quality fly ash in concrete has shown remarkable improvement in durability of concrete, especially in aggressive environment.
- Some of the technical benefits of the use of fly ash in Green Concrete are:
a) Higher ultimate strength
b) Increased durability
c) Improved workability
d) Reduced bleeding
e) Increased resistance to alkali-silica reactivity.
f) Reduced shrinkage.
7.0 Green Concrete Mix Design:
This can be done by seeing the slump test of dry all – in – aggregates and other materials. They must stand in natural angle of repose as seen in figure 12.
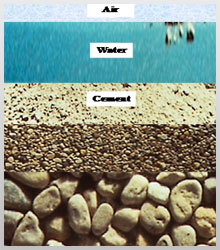
7.1 Green Concrete Mix Design Objectives:
- Optimizes void space between aggregates by optimizing particle proportions and packing of materials. This makes more effective use of the cement binder.
- Aggregates replace excess cement paste to give improved stability, less shrinkage and increase in strength & durability.
- Less cement also generates less heat of hydration.
- The slump of the concrete and its flow are a function of the shape & the quantity of the predominant size of the aggregate in the mix.
- Use of more fine aggregate gives higher slump & flow. So the optimum proportions of coarse & fine aggregate must be critically found to have the best and dense concrete in both fresh & hardened stage of concrete.
Figure 12 – Slump test of all in combined aggregate
7.2 Advantage Of Green Concrete:
It will give enhanced cohesion so user friendly – easier to place, compact & finish concrete. It can be seen in concrete slump given in figure 13. Some other advantages of such mix are:
- Optimized mix designs mean easier handling, better consistency and easier finishing
- Reduction in shrinkage & creep
- Green Concrete uses local and recycled materials in concrete.
- The heat of hydration of green concrete is significantly lower than traditional concrete
- This result in a lower temperature rise in large concrete pours which is a distinct advantage for green concrete.
Improved engineering properties:
- Mix can result in a reduced paste volume within the concrete structure resulting in a higher level of protection against concrete deterioration.
- Higher strength per kilogram of cement
- Increased durability & lower permeability
- More aggregates typically mean higher Modulus of elasticity.
Concrete stiffness or MOE is an important property of concrete in a reinforced concrete structure.
Figure 13 – Typical spread of mix – note the aggregate distribution & that it does not bleed
8.0 Geopolymer Green Concrete:
8.1 What Is Geopolymer?
Geopolymer Concrete:
Conventional concrete:
Conventional Cement + water = Hydration process gets hard product on curing
The Geopolymer paste binds the coarse and fine aggregates and other un-reacted materials together to form Geopolymer Concrete. The production of Geopolymer Concrete is similar to that of Portland Cement Concrete. In both types of concrete, the aggregates occupy the largest volume. It is truly a Green Concrete. The structural model of Geopolymeric material is still under investigation. One of the ‘visualized mechanism’ of Geopolymerisation’ is ‘dissolution, transportation and poly condensation’, which takes place through an exothermic process.
The Silicon and aluminum in the fly ash are activated by a combination of Sodium hydroxide and Sodium silicate solutions to form the Geopolymer paste that binds the aggregates and other un-reacted materials. They are defined as:
- These are also termed – ‘Alkali Activators’
- These can be single or a combination of several materials
- A combination of Sodium or Potassium Silicate and Sodium or Potassium hydroxide has been widely used
- The ‘Alkali Activator – to – base material’ (i.e., flyash or slag) ratio is generally in the range of 0.25 to 0.35
- Hydroxide solutions of Concentration 8 M to 16 M are generally used. Chemical composition of Geopolymers is similar to Zeolites, but shows an amorphous micro – structure.
8.2 Properties of fresh Geopolymer Concrete Mix:
a) Workability Of Geopolymer Concrete Mix:
- Fresh fly ash based Geopolymer concrete, in general, has good consistency and is glossy in appearance. Generally it is cohesive as seen from figure 14.
- Similar to Portland Cement Concrete, water content in the mix influences the workability, as measured by conventional slump test
- The mix is aminable for vibration
- Generally such concretes can be workable upto about 1 1/2 hrs.
b) Curing Of Geopolymer Concrete:
Curing is generally carried out at elevated temperature in the range of 50 to 80OC. Adequate humidity has to be ensured otherwise the product/element is to be insulated to preserve water in the mix.
- Curing is done generally at elevated temperatures (ensuring humidity), right from the time water and activation source material are added to the base material.
- Curing at elevated temperatures (ensuring humidity or insulation) after about 12 hours when water and activation source material are added to base material.
- Later curing is done at normal temperatures in traditional way. Duration of curing is generally for a short period; say about 3 to 5 days. Generally higher the curing temperature, higher is the compressive strength achieved
Figure 14 – Shape of Geopolymer Concrete mix Slump Cone
8.3 Properties of Hardened Geopolymer Concrete:
a. Strength Of Geopolymer Concrete:
- The Strength of Geopolymer Concrete depends on the nature of source materials and the curing temperature.
- At present Geopolymer Concrete upto 90 MPa Strength has been developed.
- Geopolymer concrete made from calcined source materials such as metakaolin (calcined kaolin), flyash, slag etc. reach higher strength when compared to those made from non-calcined materials such as Kaolin.
- The alkali activator used for Geopolymerisation also has a dominant influence on strength.
b. Stress – Strain Relations:
- The general Stress – Stress curves of Geopolymer concrete, indicate that the Stress – Strain relations are similar to that of Portland Cement Concrete.
- The Stress – Strain relations of geopolymer concrete can also be predicted using equations developed for Portland Cement Concrete.
c. Long Term Properties:
- Experiments on typical mixes have shown that fly ash based polymer concrete undergoes low creep.
8.4 Applications Geopolymer Concrete: